Nanocellulose as a Vehicle for Drug Delivery and Efficiency of Anticancer Activity: A Short-Review
DOI:
https://doi.org/10.37934/jrnn.1.1.3043Keywords:
Nanocellulose, Mechanical properties, Chemical properties, Drug delivery systems, Cancer treatment, Biomedical applicationsAbstract
With the high demand of using nanotechnology, nanocellulose has become popular for different biomedical and anticancer applications. Cellulose, a nature gifted material and the most abundant organic polymer on earth, is systematically reviewed. Details of the mechanical and chemical structure of nanocellulose are explained, starting with preparation methods along with physiochemical properties and pH gradient to incorporate innovative polymeric drug delivery vehicles in anticancer applications. A myriad of research fields has introduced nanocellulose as an intriguing candidate for anticancer drug excipient and carrier in modern cancer therapy. Albeit, innovative nanocellulose-based drug carrier systems will be complicated for their commercial use in pharmacies. Of this, it is required to understand the preparation, properties, and potential drug conjugation of nanocellulose to improve its interactions with human tissues.
Downloads
References
A. Blanco; M.C. Monte; C. Campano; A. Balea; N. Merayo; C. Negro. Nanocellulose for industrial use: Cellulose nanofibers (cnf), cellulose nanocrystals (cnc), and bacterial cellulose (bc), in Handbook of nanomaterials for industrial applications. 2018, Elsevier. p. 74-126.
N. Lin; A. Dufresne. Nanocellulose in biomedicine: Current status and future prospect. Eur. Polym. J. 2014, 59, p. 302-325. doi.org/10.1016/j.eurpolymj.2014.07.025.
M. Yusefi; R. Bte Rasit Ali; E.C. Abdullah; K. Shameli. Analysis on physiochemical properties of cellulose fiber from rice straw waste. MS&E. 2020, 808(1), p. 012038. doi.org/10.1088/1757-899X/808/1/012038.
M. Kaushik; A. Moores. Nanocelluloses as versatile supports for metal nanoparticles and their applications in catalysis. Green Chem. 2016, 18(3): p. 622-637. doi.org/10.1039/C5GC02500A
M. Österberg; E.D. Cranston. Special issue on nanocellulose. Nord Pulp Pap Res J. 2014, 29(1). doi.org/10.1039/C5GC02500A.
P.K. Gupta; S.S. Raghunath; D.V. Prasanna; P. Venkat; V. Shree; C. Chithananthan; S. Choudhary; K. Surender; K. Geetha. An update on overview of cellulose, its structure and applications, in Cellulose. 2019, IntechOpen. doi.org/10.5772/intechopen.84727.
M. Yusefi; K. Shameli; H. Jahangirian; S.-Y. Teow; H. Umakoshi; B. Saleh; R. Rafiee-Moghaddam; T.J. Webster. The potential anticancer activity of 5-fluorouracil loaded in cellulose fibers isolated from rice straw. Int. J. Nanomedicine. 2020, 15, p. 5417-5432. doi.org/10.2147/IJN.S250047.
Y. Li; H. Sun; Y. Zhang; M. Xu; S.Q. Shi. The three-dimensional heterostructure synthesis of zno/cellulosic fibers and its application for rubber composites. Compos Sci Technol. 2019, 177, p. 10-17. doi.org/10.1016/j.compscitech.2019.04.012.
K.Y. Lee; G. Buldum; A. Mantalaris; A. Bismarck. More than meets the eye in bacterial cellulose: Biosynthesis, bioprocessing, and applications in advanced fiber composites. Macromol. Biosci. 2014, 14(1), p. 10-32. doi.org/10.1002/mabi.201300298.
K.Y. Lee; G. Buldum; A. Mantalaris; A. Bismarck. More than meets the eye in bacterial cellulose: Biosynthesis, bioprocessing, and applications in advanced fiber composites. Macromol. Biosci. 2014, 14(1), p. 10-32. doi.org/10.1002/mabi.201300298.
L.A. Nor; A. Ishak. Extraction and characterization of nano cellulose from coconut fiber. Malaysian Journal of Analytical Sciences. 2013, 17(1), p. 109-118.
B.M. Cherian; A.L. Leão; S.F. De Souza; S. Thomas; L.A. Pothan; M. Kottaisamy. Isolation of nanocellulose from pineapple leaf fibres by steam explosion. Carbohydr. Polym. 2010, 81(3), p. 720-725. doi.org/10.1016/j.carbpol.2010.03.046.
P. Satyamurthy; N. Vigneshwaran. A novel process for synthesis of spherical nanocellulose by controlled hydrolysis of microcrystalline cellulose using anaerobic microbial consortium. Enzyme Microb. Technol. 2013, 52(1), p. 20-25. doi.org/10.1016/j.enzmictec.2012.09.002.
J.P.S. Morais; M. de Freitas Rosa; L.D. Nascimento; D.M. do Nascimento; A.R. Cassales. Extraction and characterization of nanocellulose structures from raw cotton linter. Carbohydr. Polym. 2013, 91(1), p. 229-235. doi.org/10.1016/j.carbpol.2012.08.010.
N. Johari; I. Ahmad; N. Halib. Comparison study ofhydrogels properties synthesized with micro-andnano-size bacterial cellulose particles extracted from nata de coco. Chem Biochem Eng Q. 2012, 26(4), p. 399-404.
J.P. Reddy; J.-W. Rhim. Characterization of bionanocomposite films prepared with agar and paper-mulberry pulp nanocellulose. Carbohydr. Polym. 2014, 110, p. 480-488. doi.org/10.1016/j.carbpol.2014.04.056.
A. Mandal; D. Chakrabarty. Isolation of nanocellulose from waste sugarcane bagasse (scb) and its characterization. Carbohydr. Polym. 2011, 86(3), p. 1291-1299. doi.org/10.1016/j.carbpol.2011.06.030.
N. Lani; N. Ngadi; A. Johari; M. Jusoh. Isolation, characterization, and application of nanocellulose from oil palm empty fruit bunch fiber as nanocomposites. J. Nanomater. 2014, 2014. doi.org/10.1155/2014/702538.
A. Dufresne; J.Y. Cavaillé; M.R. Vignon. Mechanical behavior of sheets prepared from sugar beet cellulose microfibrils. J. Appl. Polym. Sci. 1997. 64(6), p. 1185-1194. doi.org/10.1002/(SICI)1097-4628(19970509)64:6<1185::AID-APP19>3.0.CO;2-V.
X. Wu; R.J. Moon; A. Martini. Crystalline cellulose elastic modulus predicted by atomistic models of uniform deformation and nanoscale indentation. Cellulose. 2013. 20(1), p. 43-55. doi.org/10.1007/s10570-012-9823-0.
R.-L. Wu; X.-L. Wang; Y.-Z. Wang; X.-C. Bian; F. Li. Cellulose/soy protein isolate blend films prepared via room-temperature ionic liquid. Ind. Eng. Chem. Res. 2009, 48(15), p. 7132-7136. doi.org/10.1021/ie9001052.
M. Smyth; C. Rader; J. Bras; E.J. Foster. Characterization and mechanical properties of ultraviolet stimuli?responsive functionalized cellulose nanocrystal alginate composites. J. Appl. Polym. 2018, 135(7), p. 45857. doi.org/10.1002/app.45857.
Q. Cheng; S. Wang; T.G. Rials. Poly (vinyl alcohol) nanocomposites reinforced with cellulose fibrils isolated by high intensity ultrasonication. Compos. Part A Appl. Sci. Manuf. 2009, 40(2), p. 218-224. doi.org/10.1016/j.compositesa.2008.11.009.
Y. Habibi; L.A. Lucia; O.J. Rojas. Cellulose nanocrystals: Chemistry, self-assembly, and applications. Chem. Rev., 2010, 110(6), p. 3479-3500. doi.org/10.1021/cr900339w.
A. Shimotoyodome; J. Suzuki; Y. Kumamoto; T. Hase; A. Isogai. Regulation of postprandial blood metabolic variables by tempo-oxidized cellulose nanofibers. Biomacromolecules. 2011, 12(10), p. 3812-3818. doi.org/10.1021/bm2010609.
N. Lin; A. Dufresne. Surface chemistry, morphological analysis and properties of cellulose nanocrystals with gradiented sulfation degrees. Nanoscale. 2014, 6(10), p. 5384-5393. doi.org/10.1039/C3NR06761K.
M. Gama; P. Gatenholm; D. Klemm. Bacterial nanocellulose: A sophisticated multifunctional material. 2012: CRC press.
S. Jung; B.Y. Swamy; J.B. Moon; D.H. Kim; I. Chung. Anti?aids active polyrotaxane?azt conjugates with bioactive bulky stoppers and their nanoparticles. J Polym Sci A Polym Chem. 2012, 50(23), p. 4895-4901. doi.org/10.1002/pola.26317.
M. Yusefi; K. Shameli; A.F. Jumaat. Preparation and properties of magnetic iron oxide nanoparticles for biomedical applications: A brief review. Journal of Advanced Research in Materials Science. 2020, 75(1), p. 10-18. doi.org/10.37934/arms.75.1.1018.
H. Hamrayev; K. Shameli; M. Yusefi. Preparation of zinc oxide nanoparticles and its cancer treatment effects: A review paper. Journal of Advanced Research in Micro and Nano Engineering, 2020, 2(1), p. 1-11.
M. Yusefi; K. Shameli; R.R. Ali; S.-W. Pang; S.-Y. Teow. Evaluating anticancer activity of plant-mediated synthesized iron oxide nanoparticles using punica granatum fruit peel extract. J. Mol. Struct. 2020, 1204, p. 127539. doi.org/10.1016/j.molstruc.2019.127539.
D. Niedzwiecki; R.M. Hasson; H.J. Lenz; C. Ye; M. Redston; S. Ogino; C.S. Fuchs; C.C. Compton; R.J. Mayer; R.M. Goldberg. A study of thymidylate synthase expression as a biomarker for resectable colon cancer: Alliance (cancer and leukemia group b) 9581 and 89803. The oncologist 2017. 22(1), p. 107. doi.org/10.1634/theoncologist.2016-0215.
H. Fearnhead; S. Gul. Using clinical drug resistance to kill cancer cells. Drug Target Review 2017, 2(22), p. 12-14.
B. Glimelius; W. Graf; K. Hoffman; L. Påhlman; P.-O. Sjödén; A. Wennberg. General condition of asymptomatic patients with advanced colorectal cancer receiving palliative chemotherapy: A longitudinal study. Acta Oncol. 1992. 31(6), p. 645-651. doi.org/10.3109/02841869209083847.
P. Österlund; T. Ruotsalainen; K. Peuhkuri; R. Korpela; A. Ollus; M. Ikonen; H. Joensuu; I. Elomaa, Lactose intolerance associated with adjuvant 5-fluorouracil-based chemotherapy for colorectal cancer. Clin. Gastroenterol. Hepatol. 2004, 2(8), p. 696-703. doi.org/10.1016/s1542-3565(04)00293-9.
S. Srivastava; S. Mohammad; S. Gupta; A.A. Mahdi; R.K. Dixit; V. Singh; F.M. Samadi. Chemoprotective effect of nanocurcumin on 5-fluorouracil-induced-toxicity toward oral cancer treatment. Natl J Maxillofac Surg. 2018, 9(2), p. 160. doi.org/10.4103/njms.NJMS_27_18
A.P. Rokhade; N.B. Shelke; S.A. Patil; T.M. Aminabhavi. Novel hydrogel microspheres of chitosan and pluronic f-127 for controlled release of 5-fluorouracil. J. Microencapsul. 2007, 24(3): p. 274-288. doi.org/10.1080/02652040701281365.
Z. Song; E. Chen; J. Qian; J. Xu; G. Cao; W. Zhou; F. Wang; M. Chen; D. Xu; X. Wang. Serum chitinase activity prognosticates metastasis of colorectal cancer. BMC Cancer 2019, 19(1), p. 629. doi.org/10.1186/s12885-019-5834-7.
L.-K. Zhang; S. Du; X. Wang; Y. Jiao; L. Yin; Y. Zhang; Y.-Q. Guan. Bacterial cellulose based composites enhanced transdermal drug targeting for breast cancer treatment. Chem. Eng. J. 2019, 370, p. 749-759. doi.org/10.1016/j.cej.2019.03.216.
A.K. HPS; C.K. Saurabh; A. Adnan; M.N. Fazita; M. Syakir; Y. Davoudpour; M. Rafatullah; C. Abdullah; M. Haafiz; R. Dungani. A review on chitosan-cellulose blends and nanocellulose reinforced chitosan biocomposites: Properties and their applications. Carbohydr. Polym. 2016, 150, p. 216-226. doi.org/10.1016/j.carbpol.2016.05.028.
M. Sharma. Transdermal and intravenous nano drug delivery systems: Present and future, in Applications of targeted nano drugs and delivery systems. 2019, Elsevier. p. 499-550.
J.A. Meyerhardt; L. Li; H.K. Sanoff; W. Carpenter IV; D. Schrag. Effectiveness of bevacizumab with first-line combination chemotherapy for medicare patients with stage iv colorectal cancer. J. Clin. Oncol. 2012, 30(6), p. 608. doi.org/10.1200/JCO.2011.38.9650.
N. Jawahar; S. Meyyanathan. Polymeric nanoparticles for drug delivery and targeting: A comprehensive review. International Journal of Health & Allied Sciences, 2012, 1(4), p. 217. doi.org/10.4103/2278-344X.107832.
J. Bae; J.W. Park. Preparation of an injectable depot system for long-term delivery of alendronate and evaluation of its anti-osteoporotic effect in an ovariectomized rat model. Int. J. Pharm. 2015, 480(1-2), p. 37-47. doi.org/10.1016/j.ijpharm.2015.01.020.
L. Li; T.L. ten Hagen; M. Bolkestein; A. Gasselhuber; J. Yatvin; G.C. van Rhoon; A.M. Eggermont; D. Haemmerich; G.A. Koning, Improved intratumoral nanoparticle extravasation and penetration by mild hyperthermia. J. Control. Release. 2013, 167(2), p. 130-137. doi.org/10.1016/j.jconrel.2013.01.026.
Y. Matsumura; H. Maeda. A new concept for macromolecular therapeutics in cancer chemotherapy: Mechanism of tumoritropic accumulation of proteins and the antitumor agent smancs. Cancer Res. 1986. 46(12 Part 1): p. 6387-6392.
T.S. Anirudhan; J. Christa. Multi-polysaccharide based stimuli responsive polymeric network for the in vitro release of 5-fluorouracil and levamisole hydrochloride. New J Chem. 2017, 41(20), p. 11979-11990. doi.org/10.1016/j.eurpolymj.2014.07.025.
D. Zheng; Y. Deng; Y. Xia; Y. Nan; M. Peng; X. Wang; J. Yue. Fabrication and performance of a spherical cellulose nanocrystal-based hydrophobic drug delivery vehicle using rubber wood. BioResources 2019, 14(4), p. 7763-7774. doi.org/10.15376/biores.14.4.7763-7774.
K. Abe; H. Yano. Comparison of the characteristics of cellulose microfibril aggregates of wood, rice straw and potato tuber. Cellulose, 2009, 16(6), p. 1017-1023.
T.S. Anirudhan; J. Nima; P.L. Divya. Synthesis, characterization and in vitro cytotoxicity analysis of a novel cellulose based drug carrier for the controlled delivery of 5-fluorouracil, an anticancer drug. Appl. Surf. Sci. 2015, 355, p. 64-73. doi.org/10.1016/j.apsusc.2015.07.077.
J. Supramaniam; R. Adnan; N.H.M. Kaus; R. Bushra. Magnetic nanocellulose alginate hydrogel beads as potential drug delivery system. Int. J. Biol. Macromol. 2018. doi.org/10.1016/j.ijbiomac.2018.06.043.
N.A. Peppas; J.J. Sahlin. A simple equation for the description of solute release. Iii. Coupling of diffusion and relaxation. Int. J. Pharm. 1989. 57(2), p. 169-172.
R.W. Korsmeyer; R. Gurny; E. Doelker; P. Buri; N.A. Peppas. Mechanisms of solute release from porous hydrophilic polymers. Int. J. Pharm. 1983, 15(1), p. 25-35. doi.org/10.1016/0378-5173(83)90064-9.
S.K. Mallapragada; N.A. Peppas; P. Colombo. Crystal dissolution?controlled release systems. Ii. Metronidazole release from semicrystalline poly (vinyl alcohol) systems. Journal of Biomedical Materials Research: An Official Journal of The Society for Biomaterials and The Japanese Society for Biomaterials 1997. 36(1), p. 125-130. doi.org/10.1002/(sici)1097-4636(199707)36:1<125::aid-jbm15>3.0.co;2-h.
S.F. Plappert; F.W. Liebner; J. Konnerth; J.-M. Nedelec. Anisotropic nanocellulose gel–membranes for drug delivery: Tailoring structure and interface by sequential periodate–chlorite oxidation. Carbohydr. Polym. 2019, 226, p. 115306. doi.org/10.1016/j.carbpol.2019.115306.
G. Sarkar; J.T. Orasugh; N.R. Saha; I. Roy; A. Bhattacharyya; A.K. Chattopadhyay; D. Rana; D. Chattopadhyay. Cellulose nanofibrils/chitosan based transdermal drug delivery vehicle for controlled release of ketorolac tromethamine. New J. Chem. 2017, 41(24), p. 15312-15319. doi.org/10.1039/C7NJ02539D.
P. Medhi; O. Olatunji; A. Nayak; C.T. Uppuluri; R.T. Olsson; B.N. Nalluri; D.B. Das. Lidocaine-loaded fish scale-nanocellulose biopolymer composite microneedles. Aaps Pharmscitech. 2017, 18(5), p. 1488-1494. doi.org/10.1208/s12249-017-0758-5.
L. Saïdi; C. Vilela; H. Oliveira; A.J. Silvestre; C.S. Freire. Poly (n-methacryloyl glycine)/nanocellulose composites as ph-sensitive systems for controlled release of diclofenac. Carbohydr. Polym. 2017, 169, p. 357-365. doi.org/10.1016/j.carbpol.2017.04.030.
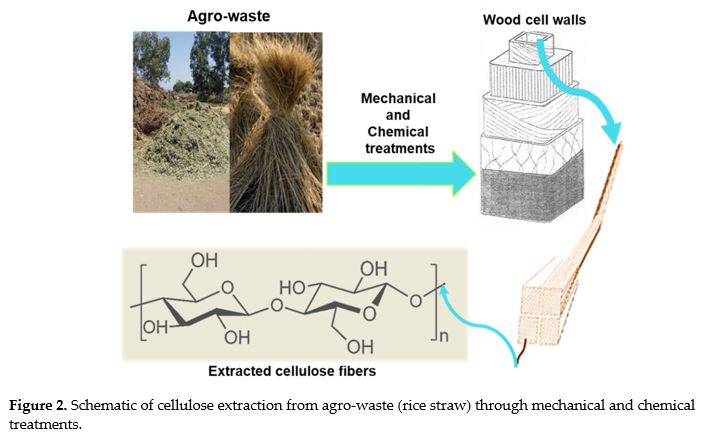